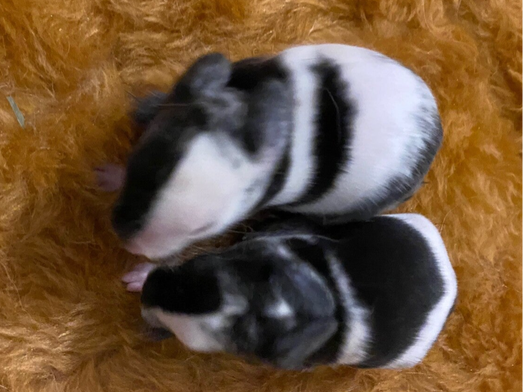
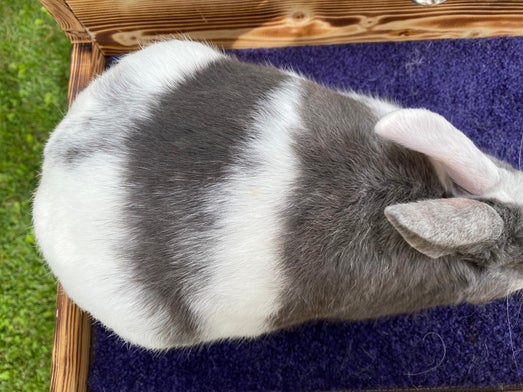
Harlequins have unique stripes and patterns unlike any other color of rabbit. But how do harlequins get their patterns? Why are the colors distributed like they are?
Two types of pigment
Before we can understand how the harlequin got its stripes, we have to first understand that mammals (including rabbits) have two different kinds of pigment: eumelanin and phaeomelanin. Eumelanin is the 'black-brown' pigment. It is responsible for making what we see as black, blue, chocolate, or lilac (depending on what other genes are involved). Phaeomelanin is the 'red-yellow' pigment. It is responsible for making what we see as red, orange, fawn, cream.
Breaking mechanisms
Normally, as fur grows in, it switches between eumelanin production and phaeomelanin production, resulting in the typical bands of an agouti color (like chestnut). In non-extension colors (like red and orange), this biological switching mechanism is broken, allowing little to no eumelanin to be produced and resulting in a rabbit with almost entirely phaeomelanin in the fur. The mutation for harlequin (eʲ) lies somewhere in the middle. It starts off as normal, but very early in development, the mechanism turns off.
So why then does the fur not grow in like non-extension, in which the eumelanin is not produced? This is because of the fact that the mechanism was working early on! As a developing embryo grows, its cells continue to divide and make new cells. This is the basis for the patchy nature of the harlequin pattern. Any cells that were in the eumelanin production phase when the switching mechanism breaks stay in that phase, and any in the phaeomelanin production phase stay in that phase. Then each cell makes new daughter cells (clones) with the broken switching mechanism stuck in whatever phase they were stuck in.
What about brindle?
The exact mechanism of what makes brindle versus harlequin is unknown. However, we know quite a bit about embryonic development and similar colors in other animals (such as tortoiseshell cats, which appear like brindles/harlequins but are due to a different gene mutation which is thought to work somewhat similarly to the harlequin mutation in rabbits). So we can think of possible ways in which this would occur. One possible explanation is timing of when the switching mechanism stops working. The later cells turn off the mechanism, the more they have already propagated around the embryo. This means that early inactivation of the switching mechanism could result in more solid patches of color, while later inactivation will result in cells more regularly interspersed between ones in the eumelanin phase and the phaeomelanin stage.
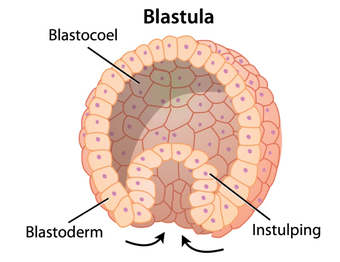
The blastula, a very early stage of the embryo, eventually divides into three layers; the endoderm, the mesoderm, and the ectoderm. The ectoderm gives rise to various tissues, including skin (and what will later form fur). Image credit
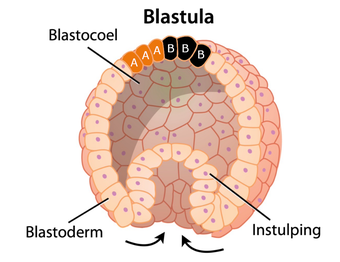
An example of how timing of the switching mechanism breakage can affect how the colored hairs form. If the mechanism is broken early in the embryonic development, before many cell lines have formed (in this case, only two; cell lines A and B), each individual cell line will give rise to more clonal cells with the same 'off position' of the mechanism. Meaning the cells will form patches.
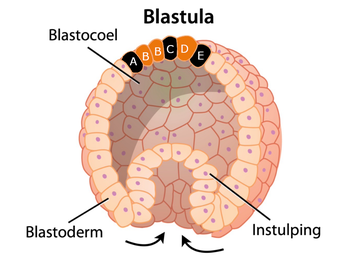
Alternatively, if the mechanism is broken later in the embryonic development, after quite a few cell lines have formed (in this case, cell lines A, B, C, D, and E), each individual cell line will have less effect on the eventual pattern, so different color hairs will be more interspersed (brindled). The timing may be influenced by genetic factors we don't yet understand.
So how do the stripes form?
Stripes and even checkerboard patterns are normal due to the way tissue develops in animals. As embryos form, tissue develops in a somewhat linear pattern along what are called the "Blaschko's lines" or "cutaneous lines of embryogenesis" (ul Bari, 2015). These are pathways of epidermal (skin) cell development and proliferation. Normally these lines are invisible. Even humans have them! Only when there's a genetic anomaly (such as a skin condition, mosaicism...or something like the harlequin mutation!) can we easily visualize them.
Various common patterns found in humans along the Blaschko's lines. Hmm, some of these look very familiar, don't they?
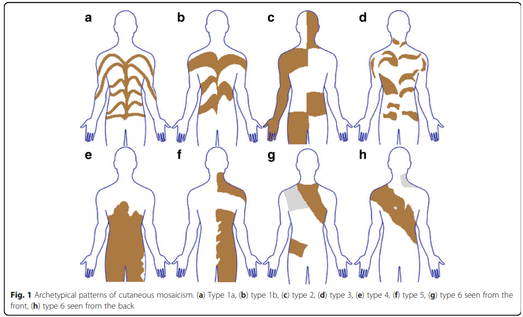
Type 1a: Narrow bands
Type 1b: Broad bands
Type 2: Checkerboard pattern (also called flag-like) characterized by alternating squares with a sharp midline separation.
Type 3: Phylloid pattern with leaf-like or oblong macules showing a dorsal and ventral midline separation.
Type 4: Patchy pattern without midline separation
Type 5: Lateralization pattern
Type 6: Sash-like pattern.
From: Kromann et al., 2018
Put it all together, and what do you get?
When you put together the broken pigment switching mechanism with the Blaschko's lines, it is easy to see how harlequin patterns could form! Early in development, the switching mechanism turns off, and each cell now is stuck in whatever phase it was in. They then divide and proliferate along the Blaschko's lines pathways, spreading their specific phase along with them.
We don't know exactly what causes the different patterns to form in humans, let alone rabbits. Why one becomes broad band and another becomes checkerboard pattern is a mystery still up for discovery! However, if the harlequin patterns are being formed along the Blaschko's lines (as they appear to be), then we can surmise that the patterns of the Blaschko's lines are somewhat genetic. Harlequin patterns are somewhat genetic. We can select for more of a checkerboard pattern, broad band patterns, or narrow band patterns, for example. While it's not perfect, selecting for one specific pattern tends to increase the chance of that pattern popping up again.
Add comment
Comments